What is CNC Turning Technology?
CNC (Computer Numerical Control) turning is a machining process where a cutting tool, typically a non-rotary tool bit, removes material from a rotating workpiece. This technology is fundamental within the broader scope of CNC machining, transforming traditional manufacturing methods by introducing precision, automation, and versatility into modern production systems.
The Core Principles of CNC Turning
CNC turning shapes materials, usually metal or plastic, by holding the workpiece on a lathe. The lathe rotates the workpiece at high speeds while a stationary cutting tool is positioned against it to perform desired cuts. The “turning” refers to the rotating motion of the workpiece, with the cutting tool remaining stationary and manipulated by the machine’s computerized control system.
The fundamental objective of CNC turning is to produce cylindrical, conical, or flat shapes. Typical products manufactured through CNC turning include various mechanical components such as shafts, bushings, bolts, and complex automotive parts like engine blocks and cylinders. It is especially beneficial when tight tolerances, high surface finish, and high repeatability are required.
CNC Turning vs. CNC Milling
CNC turning is often compared to CNC milling, another popular machining process. While CNC milling involves cutting material by rotating the tool and keeping the workpiece stationary, CNC turning does the opposite. This distinction is essential, as CNC turning is typically employed for round or cylindrical shapes, whereas CNC milling is used for more complex geometries, such as slots, holes, or irregular shapes.
Materials Used in CNC Turning
CNC turning can be applied to a variety of materials, depending on project requirements. The most common materials processed through CNC turning include metals such as steel, aluminum, brass, titanium, and harder-to-machine materials like stainless steel and high-temperature alloys. Plastics such as nylon, PVC, and acrylic are also frequently turned.
Each material presents its own challenges and advantages. Softer metals like aluminum are easier to machine but require precise control to avoid damage due to high cutting speeds. Conversely, harder metals like titanium offer greater strength but can wear out cutting tools more quickly. Proper material selection is crucial for ensuring the part can withstand its operational environment.
The Precision of CNC Turning
One of the key benefits of CNC turning is its ability to deliver high levels of precision. Advanced CNC lathes feature sophisticated control systems that allow operators to set cutting paths and dimensions down to small tolerances, often within a range of 0.025 to 0.13 mm (0.001 to 0.005 inches). This precision makes CNC turning ideal for manufacturing components requiring strict dimensional accuracy, such as aerospace and medical parts.
Accuracy is achieved through precise movement of the cutting tool and the rotational speed of the workpiece. The lathe’s spindle controls the workpiece’s rotational speed, while the computer-controlled tool holder moves the cutting tool along a pre-programmed path. This automation enables consistent, repeatable results, reducing the chance of human error and increasing overall production efficiency.
Types of CNC Turning Operations
CNC turning encompasses a variety of specific operations, each tailored to a specific purpose or shape of the workpiece. Common turning operations include:
- Straight Turning: The cutting tool moves along the length of the rotating workpiece, producing a cylindrical shape.
- Taper Turning: The tool creates a conical shape by moving at an angle to the axis of the workpiece.
- Facing: The cutting tool moves perpendicular to the axis of rotation, cutting a flat surface on the end of the workpiece.
- Thread Cutting: The tool cuts internal or external threads on the workpiece, often on parts like screws and bolts.
- Grooving: Involves cutting grooves or recesses into the workpiece for features like O-rings.
- Drilling and Boring: CNC lathes can also drill holes or bore existing holes for improved accuracy.
Each operation requires a specific toolpath and cutting tool, with the machine’s CNC programming carefully configured to meet the exact specifications of the part being manufactured.
CNC Turning Equipment
The primary machine used for CNC turning is the CNC lathe, which comes in various configurations, from basic two-axis machines to more advanced multi-axis systems. The number of axes corresponds to the degrees of freedom in which the cutting tool and workpiece can move.
- 2-Axis CNC Lathes: These offer two directions of movement: one for the workpiece’s rotation and another for the cutting tool’s longitudinal movement.
- 3-Axis CNC Lathes: These machines allow an additional axis, typically for positioning the tool at an angle to the workpiece.
- Multi-Axis CNC Lathes: More complex machines, like 4-axis or 5-axis, allow the cutting tool to move in multiple planes, enabling intricate geometries without needing to reposition the workpiece.
Modern CNC lathes often feature additional components such as automatic tool changers (ATCs), robotic arms for loading and unloading parts, and live tooling, allowing for operations like drilling or milling without moving the workpiece to another machine.
CNC Turning Process
The CNC turning process begins with a design created using CAD (Computer-Aided Design) software. This design is converted into a CNC program using CAM (Computer-Aided Manufacturing) software, consisting of a series of commands, often written in G-code, controlling the machine’s movements.
Once the program is uploaded to the CNC lathe, the operator secures the workpiece in the chuck and selects the appropriate cutting tool. The machine is calibrated, and the program is executed, rotating the workpiece at a specified speed while the cutting tool moves in a precise pattern to shape the part.
Applications of CNC Turning
CNC turning has a wide range of applications across various industries, including:
- Automotive: Producing engine components, transmission parts, and other mechanical parts requiring high precision and strength.
- Aerospace: Manufacturing components such as turbine blades, shafts, and landing gear parts due to their complex shapes and critical tolerances.
- Medical: Creating surgical instruments, implants, and other medical devices requiring high levels of accuracy and reliability.
- Electronics: Manufacturing connectors, housings, and other small components used in electronic devices.
- General Manufacturing: Producing shafts, pulleys, bushings, and other cylindrical components.
Advantages of CNC Turning
CNC turning offers numerous advantages over traditional machining methods:
- High Precision: Produces parts with extremely tight tolerances and smooth surface finishes.
- Repeatability: Allows identical parts to be produced with minimal deviation once a program is established.
- Versatility: Can handle a wide range of materials and part geometries suitable for various industries.
- Efficiency: Reduces manual intervention, speeding up production times and lowering labor costs.
- Complex Part Production: Multi-axis CNC lathes can create intricate geometries difficult or impossible to achieve with manual turning.
This overview of CNC turning technology highlights its significance in modern manufacturing, showcasing its precision, efficiency, and versatility across various applications and industries.
What is the Difference Between CNC Turning and CNC Milling?
CNC turning and CNC milling are two of the most widely used machining processes in manufacturing today. While both processes involve the removal of material to create precise parts, they differ significantly in terms of the tools used, the types of movement involved, the shapes they can produce, and the specific applications they serve. Understanding these differences is crucial for manufacturers to select the most appropriate process for their specific applications.
Basic Principles of CNC Turning vs. CNC Milling
CNC Turning is primarily used for creating cylindrical or round parts. In this process, the workpiece rotates while the cutting tool remains stationary, removing material from the outside or inside of the rotating workpiece. This method is ideal for producing symmetrical shapes like shafts, bushings, and other parts that are circular in cross-section.
CNC Milling, on the other hand, employs a rotating cutting tool that moves around a stationary workpiece. The milling tool cuts away material in multiple axes, making it suitable for creating complex shapes, flat surfaces, holes, pockets, and slots. While CNC turning focuses on primarily round parts, CNC milling(more knowledge) can produce a wider variety of shapes, including irregular geometries.
The fundamental distinction lies in the movement of the workpiece and the cutting tool:
- In CNC turning, the workpiece rotates while the tool moves along the workpiece.
- In CNC milling, the tool rotates while the workpiece remains stationary (or moves minimally).
This difference in movement creates variations in the types of parts that each process is best suited for, the tools used, and the methods of operation.
Comparison of CNC Turning and CNC Milling
To better understand the differences, here’s a comparison of key factors across both processes:
Feature | CNC Turning | CNC Milling |
---|---|---|
Workpiece Movement | Workpiece rotates, tool is stationary. | Workpiece is stationary, tool rotates. |
Primary Shape Produced | Cylindrical, conical, round parts. | Complex, flat, contoured parts. |
Best Suited For | Axles, shafts, bushings, screws. | Gears, housings, pockets, complex shapes. |
Cutting Tool | Single-point cutting tool. | Multi-point cutting tool (end mills, drills). |
Axes of Movement | Typically 2-3 axes. | 3-5 axes (more in advanced milling). |
Materials Used | Metals, plastics, and certain composites. | Metals, plastics, composites, and wood. |
Precision | High precision for round or symmetrical parts. | High precision for complex geometries. |
Typical Tolerances | ±0.001 to ±0.005 inches. | ±0.001 to ±0.005 inches (or tighter). |
Surface Finish | Smooth finish, especially on round parts. | Varied surface finishes. |
Setup Complexity | Relatively simple setup and programming. | More complex setup with multi-axis control. |
From this table, it is evident that CNC turning and CNC milling serve different purposes, with CNC turning focused on round, symmetrical parts, while CNC milling is more suited for producing complex shapes.
Types of Parts Produced by CNC Turning and CNC Milling
Parts Produced by CNC Turning:
- Cylindrical Shafts: Common in automotive and aerospace applications, requiring high precision.
- Bushings and Bearings: Benefit from the rotational accuracy that CNC turning offers.
- Screws and Bolts: Threaded components efficiently produced with precise threading.
- Conical Parts: Includes tapered bearings and rotating machine parts with varying diameters.
Parts Produced by CNC Milling:
- Complex Geometries: Such as gears with multiple curved and angled surfaces.
- Pockets and Slots: Required for internal features and detailed machining.
- Housings and Enclosures: Often needed for electronics or mechanical systems with precise cuts.
- Flat Surfaces and Profiles: Ideal for producing flat surfaces and maintaining accurate dimensions.
Tooling Differences in CNC Turning and CNC Milling
A key difference between the two processes is in the tools used.
In CNC turning, the primary tool is a single-point cutting tool designed to remove material as the workpiece rotates. The tool’s geometry is critical for achieving the desired finish and accuracy.
In CNC milling, various multi-point cutting tools are used, including:
- End Mills: For general cutting in multiple directions.
- Drills: For making holes.
- Face Mills: For creating flat surfaces.
- Ball Nose Cutters: For finishing contoured surfaces.
The variety of tools in CNC milling offers flexibility for creating intricate designs, whereas CNC turning primarily relies on single-point tools for cylindrical shapes.
Axes of Movement: CNC Turning vs. CNC Milling
The number of axes of movement varies significantly between the two processes:
- CNC Turning typically operates with 2 or 3 axes, moving along the X and Z axes for linear cuts. Advanced lathes may feature additional axes for more complex operations.
- CNC Milling machines often have 3, 4, or even 5 axes, allowing for more intricate and detailed cuts, particularly in industries that require high precision, like aerospace and medical device manufacturing.
Precision and Tolerances in CNC Turning vs. CNC Milling
Both processes offer high precision, but the specific requirements often determine which is more suitable:
- CNC Turning is typically more precise for round parts, with tolerances as tight as ±0.001 inches, making it ideal for applications like shafts and bearings.
- CNC Milling also provides high precision, especially for complex geometries, often achieving tolerances within ±0.001 to ±0.005 inches. However, the tool’s movement can introduce variations.
In many cases, the two processes are used together; for instance, a part may be turned first and then milled for added details.
Surface Finish and Material Removal Rates
Surface finish and material removal rates (MRR) differ between CNC turning and CNC milling:
- CNC Turning generally produces smoother finishes on round parts, suitable for components like bushings and shafts, with typical surface finishes ranging from Ra 0.4 to Ra 3.2 µm.
- CNC Milling can produce varied finishes depending on the tool, material, and feed rate, achieving surface finishes as fine as Ra 0.8 to Ra 6.3 µm.
Material removal rates vary:
- In CNC turning, material is consistently removed along the entire length, leading to higher MRR for cylindrical shapes.
- In CNC milling, MRR can vary based on part complexity and number of passes.
Process | Typical Surface Finish (Ra) | Material Removal Rate (cm³/min) |
---|---|---|
CNC Turning | 0.4 to 3.2 µm | 50 to 100 |
CNC Milling | 0.8 to 6.3 µm | 20 to 60 |
Applications and Industries
Both CNC turning and CNC milling are essential in various industries but are used for different applications:
- CNC Turning Applications:
- Automotive: Engine components, axles, and shafts.
- Aerospace: Rotating components like turbine shafts.
- Medical: Round implants and surgical tools.
- General Manufacturing: Bushings and rollers.
- CNC Milling Applications:
- Aerospace: Complex geometries for engine components.
- Electronics: Housings and small intricate parts.
- Medical: Custom surgical instruments and implants.
- Industrial Equipment: Gears and parts with pockets and holes.
Setup and Programming Complexity
Both CNC turning and milling require programming and setup, but CNC milling typically involves more complexity:
- CNC Turning Setup is generally simpler, as the workpiece is clamped in a chuck, and the cutting tool follows straightforward paths.
- CNC Milling Setup requires more detailed configuration due to additional axes and tool variety, often needing more extensive programming to ensure correct paths and prevent collisions.
In summary, CNC turning and CNC milling are distinct machining processes tailored to different applications, each with its own strengths and optimal use cases. Understanding these differences enables manufacturers to select the appropriate process based on their specific production needs.
What are the Core Components of CNC Turning?
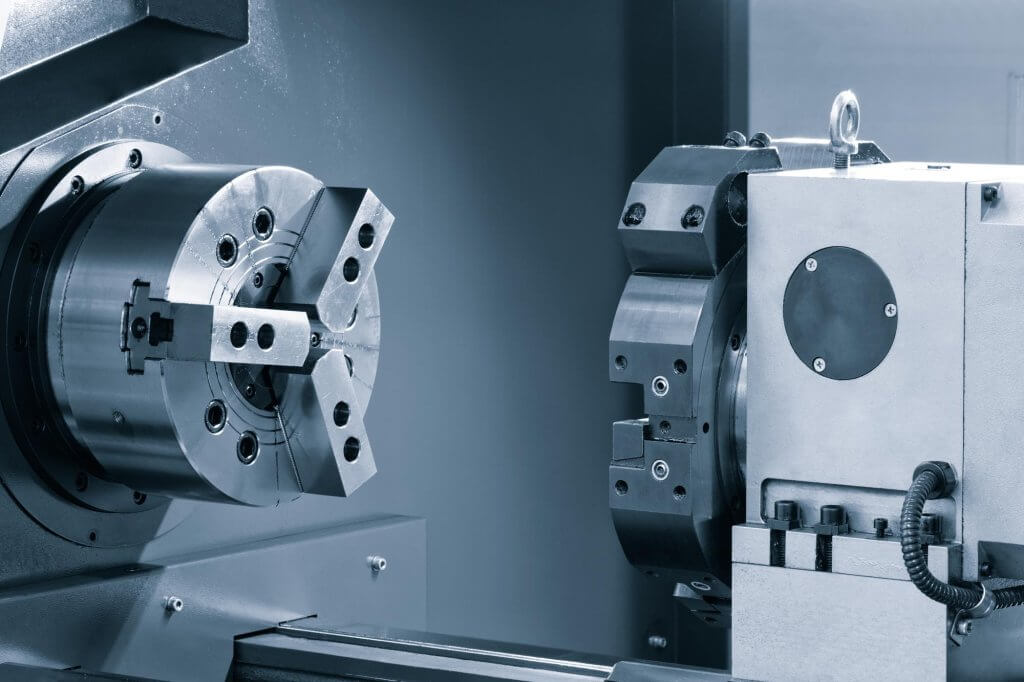
CNC turning is a highly precise and versatile manufacturing process that relies on a range of components working in harmony to create parts with exact specifications. The core components of a CNC turning machine are fundamental to its operation and performance, influencing everything from the accuracy and speed of the process to the types of materials that can be worked on. Understanding these components is essential for anyone involved in CNC machining, whether you’re a machine operator, engineer, or a business owner considering investing in CNC technology.
This chapter explores the major components of CNC turning machines, how they function together, and their impact on the machining process. These components include the CNC controller, spindle, chuck, turret, cutting tools, tailstock, and additional elements such as coolant systems, tool changers, and safety devices. We’ll also provide an overview of how these components affect machining performance, material handling, and production efficiency.
1. CNC Controller: The Brain of the Machine
At the heart of every CNC turning machine is the CNC controller. This system acts as the brain of the machine, processing programmed instructions and sending commands to the various mechanical components to perform the required operations. The CNC controller operates using a combination of hardware and software, including microprocessors, memory modules, and control software.
The controller receives input in the form of G-code, which is a programming language used in CNC machining. G-code dictates the movements of the machine, including the speed of the spindle, the feed rate of the cutting tool, and the positioning of the tool relative to the workpiece. The controller translates this code into electrical signals that guide the movement of the machine’s motors and actuators.
Key features of the CNC controller include:
- Interface Panel: Allows the operator to input commands, monitor machine performance, and make adjustments during operation.
- Display Screen: Shows real-time information about the machine’s status, including spindle speed, tool position, and part program execution.
- Memory and Storage: Stores part programs, allowing the machine to repeatedly perform the same operation without reprogramming.
- Servo Control Systems: These systems manage the precise movements of the spindle and cutting tools, ensuring high accuracy and repeatability.
The quality and sophistication of the CNC controller play a significant role in determining the performance of the machine. Advanced controllers are capable of multi-axis control, complex tool paths, and real-time adjustments based on sensor feedback, making them essential for high-precision and high-speed turning operations.
2. Spindle: The Powerhouse of CNC Turning
The spindle is another critical component of a CNC turning machine, responsible for rotating the workpiece during machining. It holds the workpiece securely in place while providing the necessary rotational speed (RPM) for the cutting tool to remove material effectively.
The spindle is driven by a motor, and its speed is adjustable based on the material being machined and the type of operation being performed. A high-speed spindle is necessary for machining softer materials or when fine surface finishes are required, while lower speeds may be needed for harder materials or roughing operations.
Key functions of the spindle include:
- Workpiece Rotation: The spindle provides the necessary torque to rotate the workpiece at speeds ranging from a few hundred to several thousand RPMs.
- Support for Tool Changes: In some CNC turning machines, the spindle is equipped with a mechanism that facilitates quick tool changes, reducing downtime between operations.
- High Precision: The spindle must maintain high levels of rotational accuracy, especially during high-speed machining, to ensure that the finished part meets the required specifications.
Advanced CNC turning machines often feature servo-driven spindles, which offer greater control over speed and positioning. These spindles can accelerate and decelerate more quickly than traditional belt-driven spindles, improving cycle times and increasing production efficiency.
3. Chuck: Securing the Workpiece
The chuck is the component responsible for holding the workpiece securely in place during the turning process. It is mounted on the spindle and grips the workpiece, allowing it to rotate while the cutting tool removes material. There are various types of chucks used in CNC turning, each designed for different types of workpieces and machining operations.
- 3-Jaw Chucks: These are the most common type of chucks, used for clamping cylindrical workpieces. They provide a balanced grip and are self-centering, which helps in aligning the workpiece with the spindle.
- 4-Jaw Independent Chucks: These chucks allow each jaw to be adjusted independently, providing more versatility for holding irregularly shaped workpieces. However, they require manual adjustment to center the workpiece.
- Collet Chucks: These chucks use collets to hold the workpiece. They provide high clamping force and accuracy, making them ideal for small-diameter parts or precision machining operations.
The choice of chuck depends on the type of material, size, and shape of the workpiece, as well as the specific requirements of the machining operation. Proper chuck selection and setup are crucial for ensuring the workpiece remains stable during turning and for achieving the desired level of precision.
Chuck Type | Best Used For | Advantages |
---|---|---|
3-Jaw Chuck | Cylindrical parts, general turning | Self-centering, quick setup |
4-Jaw Independent Chuck | Irregular shapes, precision alignment | Versatile, allows fine adjustment |
Collet Chuck | Small-diameter parts, precision work | High accuracy, excellent clamping force |
4. Turret: Tool Holding and Management
The turret is the part of a CNC turning machine that holds and indexes the cutting tools. It is mounted on a slide and moves along the X and Z axes to bring the appropriate tool into position for cutting. In modern CNC turning machines, the turret is often automated, allowing it to rotate and change tools quickly based on the machining program.
A turret typically holds multiple tools, allowing the machine to perform a variety of operations—such as turning, facing, threading, and drilling—without needing to stop and manually change tools. This versatility is key to CNC turning’s efficiency, especially in high-production environments where minimizing downtime is critical.
Some key features of the turret include:
- Tool Indexing: The turret can rotate to bring the required tool into position automatically. This reduces the time needed to switch between tools during an operation.
- Tool Holders: Tools are mounted in specialized holders that can be quickly inserted and removed from the turret. These holders are designed to provide stability and minimize vibration during cutting operations.
- Live Tooling: In some CNC turning centers, the turret is equipped with live tooling, which allows for milling, drilling, and tapping operations to be performed while the workpiece is still in the chuck. This eliminates the need to transfer the workpiece to a milling machine for secondary operations, increasing overall efficiency.
The number of tool stations on a turret can vary from machine to machine, with some turrets holding as many as 12 or more tools. The more tools that a turret can hold, the fewer manual interventions are required during the machining process, leading to higher productivity.
5. Tailstock: Support for Long Workpieces
The tailstock is a support device used in CNC turning to hold the other end of long workpieces, preventing them from deflecting or vibrating during machining. The tailstock is mounted opposite the spindle on the lathe bed and can be moved along the Z-axis to accommodate different workpiece lengths.
The tailstock is particularly important when machining long, slender parts, such as shafts or rods, that might otherwise bend or deform under the pressure of the cutting tool. The tailstock provides additional support by holding a center or a live center that is inserted into the workpiece’s end, keeping it stable and aligned with the spindle.
There are two main types of tailstocks:
- Fixed Tailstocks: These are manually adjusted and locked into place once the workpiece is secured.
- Programmable Tailstocks: These are controlled by the CNC system and can move automatically into position based on the part program.
By supporting the workpiece at both ends, the tailstock helps maintain machining accuracy and surface finish, especially during high-speed operations or when working with longer materials.
6. Cutting Tools: The Essential Elements of Material Removal
The cutting tools used in CNC turning are one of the most critical components in the entire machining process. These tools are responsible for removing material from the workpiece and must be selected based on the type of material being machined, the desired surface finish, and the specific operation being performed.
Common types of cutting tools used in CNC turning include:
- Turning Tools: Used for removing material along the workpiece’s outer surface, creating cylindrical shapes.
- Facing Tools: Used to produce flat surfaces on the ends of the workpiece.
- Threading Tools: Designed to cut internal or external threads.
- Grooving Tools: Used for creating grooves or recesses on the workpiece.
- Boring Bars: Used to enlarge or finish existing holes inside the workpiece.
Cutting tools are typically made from high-speed steel (HSS), carbide, or ceramics, with carbide being the most commonly used material due to its superior hardness and wear resistance. Proper tool selection and maintenance are crucial for maximizing tool life, improving surface finish, and achieving tight tolerances.
Cutting Tool Type | Primary Function | Best Suited For |
---|---|---|
Turning Tools | Remove material from the exterior | Cylindrical parts, general turning |
Facing Tools | Create flat surfaces | End-facing operations, precise end cuts |
Threading Tools | Cut internal/external threads | Bolts, screws, fasteners |
Grooving |
Tools | Create grooves or recesses | O-ring seats, channels, and rings |
| Boring Bars | Enlarge/finish internal holes | Precision boring, creating smooth inner walls |
7. Coolant System: Managing Heat and Chip Removal
The coolant system plays a vital role in CNC turning by controlling heat generation during cutting and ensuring efficient chip removal. High temperatures generated by friction between the cutting tool and workpiece can lead to tool wear, thermal expansion, and poor surface finish. A properly functioning coolant system mitigates these issues by cooling the cutting area and flushing chips away from the tool and workpiece.
Common coolants used in CNC turning include:
- Water-based Coolants: These are used for general-purpose machining and are effective at reducing heat.
- Oil-based Coolants: These provide better lubrication and are often used for harder materials or in high-speed operations.
- Mist Coolants: These are sprayed directly onto the cutting zone, reducing the amount of liquid used while still providing adequate cooling.
By ensuring proper cooling and chip removal, the coolant system helps to extend tool life, improve surface finish, and maintain machining accuracy.
8. Additional Components and Safety Features
Modern CNC turning machines are equipped with several additional components that enhance their functionality and ensure safe operation. These include:
- Automatic Tool Changers (ATC): These devices allow the machine to switch between different cutting tools automatically, reducing downtime and increasing productivity.
- Chip Conveyors: These systems automatically remove chips from the machining area, preventing them from interfering with the cutting process.
- Safety Enclosures: CNC turning machines are often equipped with protective enclosures that shield operators from flying chips, coolant spray, and rotating components.
These features contribute to the efficiency, safety, and reliability of CNC turning machines, making them indispensable in high-production environments.
Which Materials are Best Suited for CNC Turning?
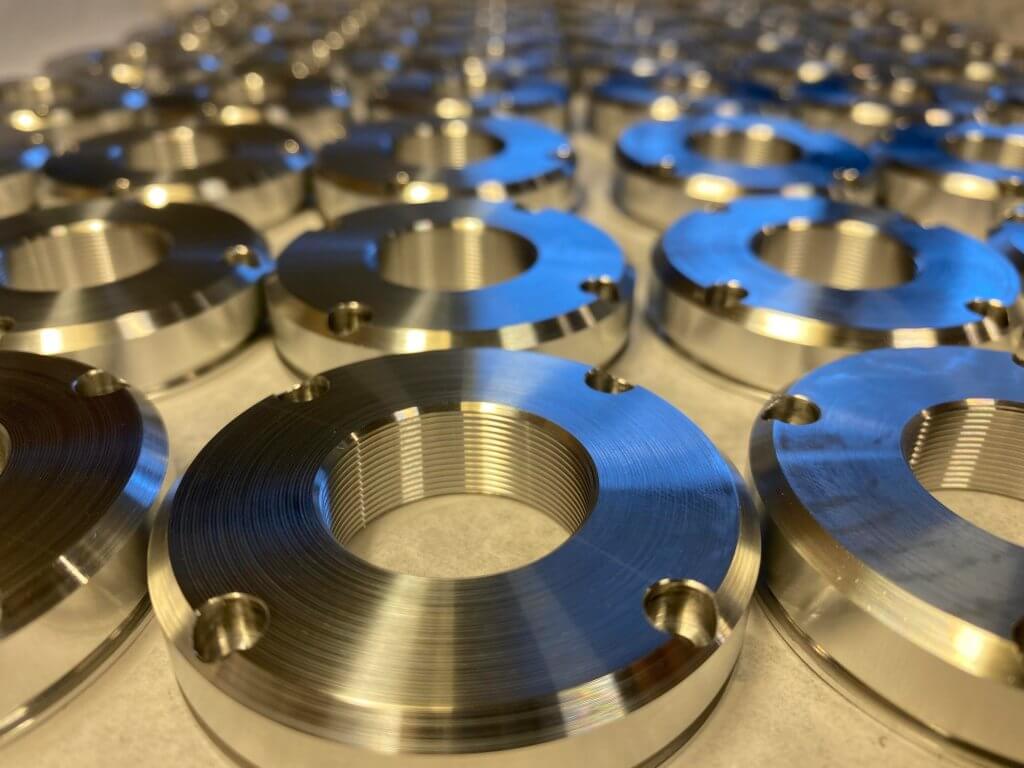
CNC turning is a versatile machining process used to manufacture parts from a wide variety of materials. The choice of material is critical in determining the final quality, functionality, and cost of the turned part. The properties of the material, such as hardness, machinability, and thermal conductivity, significantly impact how the material behaves during the turning process, influencing factors like tool wear, surface finish, and production speed.
In this chapter, we’ll explore the most common materials used in CNC turning, discussing their characteristics, advantages, disadvantages, and typical applications. The selection of material for CNC turning depends on factors such as mechanical properties, ease of machining, cost, and the end-use environment of the component.
1. Metals Commonly Used in CNC Turning
Metals are by far the most frequently turned materials in CNC operations, ranging from soft metals like aluminum to harder alloys like titanium. Each metal has its own unique characteristics that affect its suitability for different types of turned parts.
Aluminum
Aluminum is one of the most popular materials for CNC turning due to its excellent machinability, light weight, and corrosion resistance. It is soft compared to other metals, which makes it easy to machine at high speeds with minimal tool wear. Aluminum’s good thermal conductivity and strength-to-weight ratio make it ideal for automotive, aerospace, and consumer electronics applications.
- Advantages:
- High machinability
- Lightweight and corrosion-resistant
- Excellent thermal conductivity
- Good surface finish can be achieved
- Affordable compared to other metals
- Disadvantages:
- Lower strength compared to steel or titanium
- Prone to galling and scratching during machining
- Applications:
- Automotive parts like engine components
- Aerospace structures
- Electronic housings and heat sinks
Steel
Steel is another commonly used material in CNC turning, valued for its strength, durability, and availability in various grades. Steel alloys vary greatly in hardness and toughness, ranging from low-carbon steels to high-strength tool steels. Different types of steel require different machining approaches, with softer steels being easier to machine than hardened alloys. However, steel is generally more difficult to machine than aluminum, and higher tool wear and cutting forces are expected.
- Advantages:
- High strength and durability
- Available in numerous grades to suit various applications
- Good dimensional stability and resistance to deformation
- Disadvantages:
- More challenging to machine compared to softer metals
- Higher tool wear and cutting forces
- Susceptible to rusting without proper treatment (e.g., coating or galvanizing)
- Applications:
- Structural components in construction and machinery
- Automotive parts like gears, shafts, and axles
- Fasteners and hardware
Steel Grade | Characteristics | Typical Applications |
---|---|---|
Low-carbon steel | High ductility, easy to machine | Bolts, fasteners, low-stress components |
High-carbon steel | Harder, more wear-resistant | Cutting tools, high-stress parts |
Stainless steel | Corrosion-resistant, durable | Medical devices, food processing, marine components |
Stainless Steel
Stainless steel is a popular choice for CNC turning due to its corrosion resistance, strength, and aesthetic finish. It contains chromium, which forms a protective layer against rust and oxidation. While stainless steel is more difficult to machine than aluminum or mild steel, its strength and resistance to environmental factors make it ideal for parts that require longevity and exposure to harsh conditions.
- Advantages:
- Excellent corrosion resistance
- High strength and durability
- Aesthetic surface finish
- Good toughness and impact resistance
- Disadvantages:
- Harder to machine than aluminum or mild steel
- Higher costs associated with material and tool wear
- Generates significant heat during machining
- Applications:
- Medical devices and surgical instruments
- Marine and chemical processing equipment
- Food industry components (e.g., fittings and valves)
Brass
Brass is an alloy primarily composed of copper and zinc, and it is well known for its excellent machinability, corrosion resistance, and attractive appearance. Brass is softer than steel and stainless steel, making it relatively easy to machine, and it is often used when a smooth finish and intricate detail are required. Due to its aesthetic appeal and resistance to tarnishing, brass is commonly used in decorative applications.
- Advantages:
- Outstanding machinability
- Excellent corrosion resistance, especially in marine environments
- High electrical conductivity
- Attractive surface finish
- Disadvantages:
- Softer than steel and may deform under high-stress conditions
- Not suitable for high-temperature applications
- Applications:
- Electrical connectors and terminals
- Decorative components like hardware and fittings
- Plumbing components
Copper
Copper is valued for its high electrical and thermal conductivity, making it a popular material for electrical and electronic applications. While copper is relatively easy to machine, its softness can lead to issues like burr formation and poor surface finishes if not machined properly. However, with the right tool and speed settings, copper can produce high-quality turned parts.
- Advantages:
- Excellent electrical and thermal conductivity
- Easy to machine with the right parameters
- Good corrosion resistance
- Disadvantages:
- Prone to deformation due to its softness
- Can be expensive compared to other metals like aluminum
- Applications:
- Electrical components (e.g., connectors, terminals)
- Heat exchangers and radiators
- Plumbing components
Metal | Machinability | Strength | Corrosion Resistance | Common Applications |
---|---|---|---|---|
Aluminum | Excellent | Moderate | High | Aerospace parts, electronics, automotive |
Steel | Good | High | Low to Moderate | Gears, shafts, structural components |
Stainless Steel | Fair | High | High | Medical devices, marine equipment |
Brass | Excellent | Moderate | High | Electrical components, decorative parts |
Copper | Good | Moderate | High | Electrical connectors, heat exchangers |
Titanium
Titanium is highly valued in industries like aerospace, medical, and automotive for its excellent strength-to-weight ratio and corrosion resistance. However, titanium is one of the more challenging materials to machine due to its hardness and the fact that it tends to generate significant heat during machining. Specialized tools and machining strategies are required to work with titanium, but the results are worth it for applications that demand high performance.
- Advantages:
- High strength-to-weight ratio
- Excellent corrosion resistance
- Biocompatible, making it ideal for medical implants
- Disadvantages:
- Difficult to machine, requiring specialized tools
- High material and machining costs
- Generates significant heat during cutting, leading to rapid tool wear
- Applications:
- Aerospace components like turbine blades and structural parts
- Medical implants such as joint replacements and bone screws
- Automotive performance parts
2. Plastics Used in CNC Turning
CNC turning isn’t limited to metals. Plastics are also commonly turned to create lightweight, corrosion-resistant, and cost-effective parts. Plastics can be easily machined into complex shapes, making them ideal for a wide range of industries, including electronics, medical devices, and consumer goods.
ABS (Acrylonitrile Butadiene Styrene)
ABS is a versatile plastic known for its toughness, impact resistance, and machinability. It is commonly used in applications where parts need to be durable and withstand rough handling. ABS is relatively easy to machine and can be turned into parts with intricate details.
- Advantages:
- Good machinability
- High impact resistance
- Lightweight and cost-effective
- Disadvantages:
- Lower heat resistance compared to other plastics
- Not as rigid as some other engineering plastics
- Applications:
- Consumer electronics housings
- Automotive components
- Prototyping
Nylon
Nylon is a strong, durable plastic that is often used in applications requiring wear resistance and low friction. Nylon has good machinability and can produce smooth surface finishes when turned. It is commonly used in the production of gears, bearings, and other components that require low-friction operation.
- Advantages:
- Excellent wear and abrasion resistance
- Low friction
- Good toughness and impact resistance
- Disadvantages:
- Absorbs moisture, which can affect dimensional stability
- Lower heat resistance than some other plastics
- Applications:
- Gears and bearings
- Bushings and spacers
- Mechanical components
Delrin (Acetal)
Delrin, or acetal, is a high-performance plastic known for its strength, rigidity, and low friction. It is widely used in precision parts that require tight tolerances and a low coefficient of friction. Delrin’s machinability is excellent, making it one of the most popular plastics for CNC turning.
- Advantages:
- Excellent machinability
- High strength and rigidity
- Low friction and wear resistance
- Disadvantages:
- Lower impact resistance compared to ABS or nylon
- Susceptible to chemicals and solvents
- Applications:
- Precision gears and cams
- Bearings and bushings
- Electrical insulators
Polycarbonate
Polycarbonate is a tough, transparent plastic that combines high impact resistance
with excellent optical clarity. It is more difficult to machine than ABS or nylon due to its hardness, but it is widely used in applications that require both strength and transparency.
- Advantages:
- High impact resistance
- Excellent optical clarity
- Strong and durable
- Disadvantages:
- More challenging to machine than other plastics
- Susceptible to scratching
- Applications:
- Transparent machine guards
- Lenses and optical components
- Protective covers
Plastic | Machinability | Impact Resistance | Friction Resistance | Common Applications |
---|---|---|---|---|
ABS | Excellent | High | Moderate | Prototyping, housings, automotive |
Nylon | Good | High | Excellent | Gears, bearings, mechanical parts |
Delrin (Acetal) | Excellent | Moderate | High | Precision gears, electrical parts |
Polycarbonate | Fair | High | Moderate | Transparent covers, lenses |
3. Advanced Composites for CNC Turning
In addition to metals and plastics, advanced composites are increasingly being used in CNC turning for specialized applications. Composites combine two or more materials to achieve superior properties, such as high strength, low weight, and resistance to corrosion or wear. The most common composites used in CNC turning include carbon fiber-reinforced polymers (CFRP) and glass fiber-reinforced polymers (GFRP).
Carbon Fiber-Reinforced Polymers (CFRP)
CFRP is a lightweight, high-strength composite made by reinforcing a polymer matrix with carbon fibers. It is used in applications that require high performance and low weight, such as aerospace, automotive, and sporting goods. CNC turning of CFRP requires specialized tools due to its abrasive nature, but it can produce highly precise and durable parts.
- Advantages:
- Extremely high strength-to-weight ratio
- Excellent fatigue and corrosion resistance
- Lightweight
- Disadvantages:
- Difficult to machine due to its abrasive nature
- Expensive material and tooling costs
- Applications:
- Aerospace components
- High-performance automotive parts
- Sporting goods (e.g., bike frames, tennis rackets)
Glass Fiber-Reinforced Polymers (GFRP)
GFRP is similar to CFRP but uses glass fibers as the reinforcement material. It is less expensive than carbon fiber and provides good strength and durability, making it suitable for a wide range of industrial applications. GFRP is also easier to machine than CFRP, but it still requires careful attention to tool wear due to its abrasive properties.
- Advantages:
- Good strength and durability
- More affordable than CFRP
- Corrosion and wear resistance
- Disadvantages:
- Can be challenging to machine without proper tools
- Not as lightweight as CFRP
- Applications:
- Industrial piping and tanks
- Marine components
- Structural parts in construction
How Does CNC Turning Achieve High Precision Machining?
CNC turning has become the backbone of many manufacturing industries due to its ability to produce high-precision parts consistently and efficiently. Precision is the defining characteristic of CNC turning, especially when compared to traditional machining methods. High precision is essential in industries such as aerospace, medical device manufacturing, automotive, and electronics, where even minor deviations from the design can lead to failures, decreased performance, or unsafe products.
Achieving high-precision machining in CNC turning is not a simple task. It involves the integration of advanced machine components, precise control over the machining process, the use of appropriate materials, and proper maintenance and calibration. This chapter will explore how CNC turning achieves such a high level of precision and the factors contributing to its success, including the CNC system, machine rigidity, tool selection, thermal stability, and the use of modern software and monitoring systems.
1. The Role of CNC Control Systems in Precision
The most critical element in achieving high precision in CNC turning is the CNC control system itself. A CNC machine’s controller translates the design files into precise movements by controlling the machine’s motors, cutting tools, and workpiece positioning with high accuracy.
Modern CNC control systems use G-code programming to ensure that the cutting tools move in exactly the right way according to the design specifications. These systems can handle multiple axes of movement and manage complex tool paths with high precision. The advanced computing power in modern CNC systems allows for real-time corrections based on sensor feedback, which is essential in maintaining tight tolerances.
Key features of CNC control systems that contribute to high precision include:
- Servo Motors: Servo motors provide precise control over the movement of the cutting tool and the workpiece. These motors allow the machine to make quick and exact adjustments, enabling high accuracy in every pass.
- Feedback Loops: Closed-loop control systems continuously monitor the position of the tool and workpiece, making real-time adjustments to correct any deviations. This ensures that the actual position matches the programmed coordinates, resulting in precise cuts.
- Adaptive Control: Many advanced CNC machines come with adaptive control systems that automatically adjust the cutting parameters based on real-time data. For example, if the machine detects excessive tool wear or increased cutting forces, it can slow down or change the tool’s path to ensure consistent results without compromising precision.
With the right control systems in place, CNC turning machines can consistently achieve tolerances within microns, even during long production runs.
2. Machine Rigidity and Structural Integrity
The rigidity of the CNC turning machine is a major factor influencing precision. In machining, any unwanted movement or vibration can lead to inaccuracies in the final part, so maintaining a solid and stable structure is essential. Modern CNC turning machines are designed with rigid structures to minimize vibration and deflection during the machining process.
Key elements of machine rigidity include:
- Frame and Bed: The machine’s frame and bed are typically made from cast iron or other high-strength materials that provide stability and absorb vibrations. These components are designed to support heavy workpieces and cutting forces without deforming.
- Guides and Rails: Linear guides and ball-bearing rails ensure smooth and accurate movement of the machine’s slides and turret. High-precision CNC machines often use hardened and ground rails to minimize wear and maintain accuracy over time.
- Spindle Design: The spindle is the component that holds and rotates the workpiece, and it must maintain precise rotational accuracy. Modern spindles are designed with minimal runout (the deviation from perfect concentricity) and are supported by high-precision bearings to ensure stability during high-speed turning.
- Vibration Damping: Advanced CNC turning machines are equipped with vibration-damping materials and structures that minimize any unwanted oscillations during the cutting process. Reducing vibration ensures that the tool remains stable, leading to a more precise cut.
Key Structural Component | Role in Precision |
---|---|
Machine Bed and Frame | Provides stability and absorbs vibrations |
Linear Guides and Rails | Ensures smooth, accurate movement of components |
Spindle Design | Maintains rotational accuracy with minimal runout |
Vibration Damping | Reduces unwanted oscillations for a more stable cut |
Without a rigid structure, even the most advanced CNC control systems would struggle to achieve high precision, as any movement or deflection in the machine would compromise the accuracy of the cuts.
3. Precision Tooling and Tool Holders
High precision in CNC turning is also dependent on the tools and tool holders used during the machining process. Choosing the right cutting tools, maintaining them, and using precision tool holders are crucial in achieving tight tolerances and excellent surface finishes.
Cutting Tools: The selection of cutting tools is crucial for achieving high precision in CNC turning. Tools made from carbide, ceramics, or polycrystalline diamond (PCD) are commonly used in precision applications due to their hardness and resistance to wear.
- Carbide Tools: Carbide tools are favored for their high wear resistance and ability to maintain a sharp cutting edge at high temperatures. This makes them ideal for high-precision machining of harder materials like steel and titanium.
- Ceramic Tools: These tools are extremely hard and can withstand high temperatures, making them suitable for high-speed operations that require tight tolerances. However, ceramic tools are more brittle than carbide, so they are typically used for finishing operations where tool rigidity and minimal deflection are critical.
- PCD Tools: Polycrystalline diamond tools are used for machining non-ferrous materials like aluminum and plastics. These tools can achieve extremely fine surface finishes and are ideal for high-precision machining where tight tolerances are required.
Tool Holders: Tool holders play a vital role in maintaining precision by securely holding the cutting tool and minimizing any movement or vibration. High-precision tool holders are designed to provide stability and repeatability, ensuring that the cutting tool remains in the exact position during each pass. Key aspects of precision tool holders include:
- Runout Tolerance: The tool holder must have minimal runout to ensure that the cutting edge is always positioned correctly relative to the workpiece. Even a small amount of runout can lead to inaccuracies in the final part.
- Stiffness: A stiff tool holder prevents any deflection or bending during cutting, which is essential for maintaining tight tolerances, especially during high-speed operations.
- Vibration Damping: Precision tool holders often incorporate vibration-damping materials or mechanisms to reduce tool chatter and improve surface finish.
By using high-quality cutting tools and precision tool holders, CNC turning machines can achieve the fine tolerances and surface finishes required in industries like aerospace, medical devices, and automotive components.
4. Thermal Stability in CNC Turning
One of the most critical factors affecting precision in CNC turning is thermal stability. Heat is generated during machining due to the friction between the cutting tool and the workpiece. This heat can cause both the workpiece and the machine components to expand, leading to inaccuracies in the final dimensions of the part.
There are several strategies used in CNC turning to manage heat and maintain thermal stability:
- Coolant Systems: Coolants are used to reduce the heat generated during cutting and keep both the tool and the workpiece at a consistent temperature. This helps prevent thermal expansion and ensures that the part remains within the required tolerances.
- Toolpath Optimization: By optimizing the toolpath and cutting parameters, CNC programmers can minimize heat generation and reduce the likelihood of thermal deformation. This involves adjusting the feed rate, spindle speed, and depth of cut to balance material removal with heat dissipation.
- Thermal Compensation: Many modern CNC turning machines come equipped with thermal compensation systems. These systems use sensors to monitor the temperature of the machine components and adjust the cutting parameters in real-time to compensate for thermal expansion or contraction.
- Stable Machine Environment: CNC machines should be housed in temperature-controlled environments to minimize the effects of thermal fluctuations. Keeping the ambient temperature stable helps maintain consistent machining conditions, reducing the risk of dimensional inaccuracies caused by temperature changes.
In high-precision applications, controlling the thermal environment is essential to achieving consistent results, especially when machining materials that are highly sensitive to temperature changes, such as aluminum or titanium.
5. Advanced Software and Simulation Tools
Another key factor in achieving high precision in CNC turning is the use of advanced software and simulation tools. These tools allow manufacturers to model the machining process before actual production begins, identifying potential issues and optimizing the process for precision.
CAD/CAM Software: Computer-aided design (CAD) and computer-aided manufacturing (CAM) software are used to create detailed part designs and generate the necessary toolpaths for machining. These software programs allow engineers to design complex geometries with precision and simulate the machining process to ensure that the toolpaths are optimized for accuracy.
- Collision Detection: CAM software can simulate the entire machining process, including tool movements and material removal, to check for potential collisions between the tool and workpiece. This ensures that the toolpaths are safe and accurate, preventing errors that could affect the precision of the final part.
- Toolpath Optimization: By simulating the machining process, CAM software can identify the most efficient toolpaths for material removal. This reduces the number of passes required and minimizes the risk of errors caused by tool deflection or vibration.
Digital Twin Technology: Digital twin technology is an emerging trend in precision machining that involves creating a virtual replica of the CNC machine and workpiece. This digital model is continuously updated with real-time data from the machine, allowing engineers to monitor the machining process and make adjustments to ensure precision.
- Real-time Monitoring: Sensors on the CNC machine provide data on tool wear, cutting forces, temperature, and vibration, which is fed into the digital twin model. This allows manufacturers to make real-time adjustments to the machining process, ensuring that the part remains within the required tolerances.
- Predictive Maintenance: By analyzing data from the digital twin, manufacturers can predict when machine components or cutting tools are likely to fail and perform maintenance before any issues arise. This helps maintain machine accuracy and prevents unexpected downtime.
By leveraging advanced software and simulation tools, manufacturers can optimize their CNC turning operations for precision, reduce the risk of errors, and ensure that each part meets the required specifications.
6. Quality Control and Inspection in CNC Turning
Achieving high precision in CNC turning also requires rigorous quality control and inspection processes. Even with the most advanced machines and tooling, it’s essential to verify that the final parts meet the required tolerances and specifications.
In-process Monitoring: Many modern CNC turning machines are equipped with in-process monitoring systems that track key parameters such as tool wear, cutting forces, and surface finish during machining. This real-time data allows operators to make adjustments on the fly, ensuring that precision is maintained throughout the production process.
Post-production Inspection: After the parts are machined, they must undergo thorough inspection to ensure that they meet the specified tolerances. Common inspection methods include:
- Coordinate Measuring Machines (CMMs): CMMs use probes to measure the dimensions of a part with extreme accuracy. These machines can automatically check for deviations from the design and provide detailed reports on the part’s dimensional accuracy.
- Laser Scanning: Laser scanners create a 3D model of the part by capturing its surface geometry. This model is then compared to the original CAD design to identify any discrepancies.
- Optical and Tactile Probing: Some machines use optical or tactile probes to measure the part’s surface finish and dimensional accuracy while the part is still on the machine. This allows for immediate feedback and corrections if necessary.
By incorporating both in-process monitoring and post-production inspection, manufacturers can ensure that their CNC turning operations consistently produce high-precision parts.
Can CNC Turning Be Used for Complex Parts?
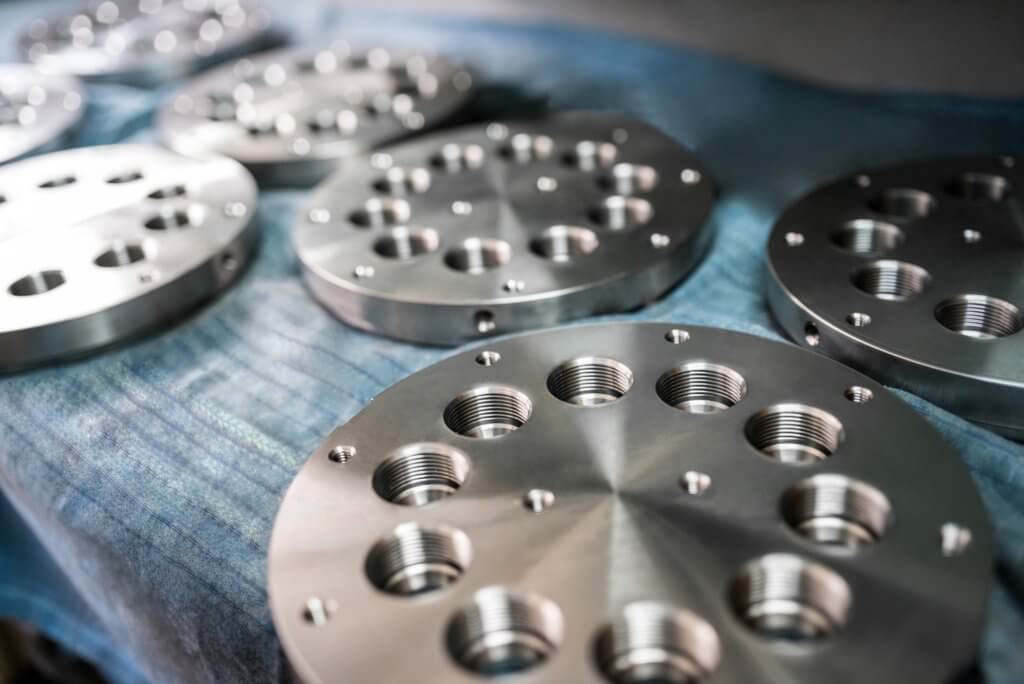
CNC turning is traditionally associated with the production of simple, symmetrical components, such as shafts, bolts, and bushings. However, advancements in CNC technology, combined with sophisticated tooling and machine configurations, have expanded the capabilities of CNC turning far beyond simple geometries. Today, CNC turning can be used to manufacture highly complex parts with intricate features and tight tolerances.
This chapter will explore the feasibility and effectiveness of CNC turning in the production of complex parts. We will discuss the factors that enable CNC turning to handle complex geometries, including multi-axis turning centers, live tooling, advanced software, and materials compatibility. Additionally, we’ll review the challenges and best practices in CNC turning for complex part manufacturing.
1. Multi-Axis CNC Turning for Complex Geometries
Traditional CNC lathes typically operate with two linear axes (X and Z) to machine cylindrical parts with rotational symmetry. While this setup is sufficient for simpler parts, it falls short when dealing with complex geometries that require non-symmetrical or multi-faceted features. However, modern CNC turning centers are equipped with multi-axis capabilities that significantly enhance their ability to produce complex parts.
- Three-Axis and Four-Axis CNC Turning: With the addition of a Y-axis and a rotating C-axis, CNC turning centers can perform milling and drilling operations in addition to turning. This allows for the machining of off-center holes, slots, and flats, enabling the production of more intricate components without the need for secondary machining processes.
- Five-Axis CNC Turning: In advanced five-axis turning, the machine can simultaneously control movement along five different axes, allowing for the creation of complex geometries, curved surfaces, and angled features. This multi-axis capability reduces the need for repositioning the workpiece, leading to increased precision and faster cycle times for complex parts.
For example, aerospace components such as turbine blades, medical implants, and high-performance automotive parts often feature complex profiles, varying radii, and undercuts that require multi-axis machining. Multi-axis CNC turning machines can handle these requirements by combining turning with milling, drilling, and tapping operations in a single setup.
2. Live Tooling for Complex Part Features
One of the most significant innovations in CNC turning is the introduction of live tooling. Live tooling refers to the use of powered tools that can perform milling, drilling, tapping, and other machining operations while the workpiece is being turned. This capability enables CNC lathes to go beyond simple rotational cuts and introduce additional complexity into the part.
Key advantages of live tooling include:
- Multiple Machining Operations: With live tooling, CNC turning machines can perform operations such as creating flat surfaces, angled features, holes, keyways, and threads directly on the lathe. This reduces the need to transfer parts between different machines, which can introduce errors and increase production time.
- Complex Part Geometry: Live tooling allows CNC turning centers to produce parts with intricate geometries that would typically require multiple setups or different machines. By integrating turning and milling operations, manufacturers can achieve highly detailed components in a single setup.
- Tighter Tolerances: Since the part remains in the same fixture throughout multiple operations, live tooling helps maintain tighter tolerances. This reduces errors that may arise from repositioning the workpiece or transferring it to other machines.
For instance, in the production of high-precision components such as valve bodies, medical instruments, and intricate automotive parts, live tooling enables the creation of complex features such as grooves, cross-holes, and angled surfaces—all without removing the part from the machine.
3. CNC Turning Centers with Sub-Spindles
To further enhance the ability of CNC turning machines to handle complex parts, many machines are equipped with sub-spindles. A sub-spindle is a secondary spindle that allows the workpiece to be transferred from the main spindle to the sub-spindle, enabling additional operations to be performed on the opposite side of the part without manual intervention.
- Improved Part Complexity: By utilizing both the main and sub-spindle, CNC turning centers can machine all sides of the part, including internal features that would be difficult or impossible to access in a single setup. This capability is particularly useful for parts with intricate internal geometries or features that require machining from multiple angles.
- Increased Productivity: The use of a sub-spindle eliminates the need to remove the part from the machine for secondary operations, improving cycle times and reducing the risk of handling-related errors.
- Enhanced Precision: Since the part is not removed from the machine during the transfer from the main to the sub-spindle, this approach maintains the part’s alignment and ensures higher precision in the final product.
For complex parts such as hollow shafts, gears with internal splines, and components with detailed internal features, the use of sub-spindles in CNC turning centers allows for complete machining in a single setup, ensuring both precision and efficiency.
4. CAD/CAM Software and CNC Programming for Complex Parts
To achieve high levels of complexity in CNC turning, manufacturers rely on advanced CAD/CAM software to design the part and program the machine. Modern CAD (Computer-Aided Design) and CAM (Computer-Aided Manufacturing) software enable the creation of intricate part geometries and generate the necessary toolpaths to machine them efficiently.
- Complex Part Design: CAD software allows engineers to create highly detailed 3D models of complex parts, complete with all the necessary features, such as holes, grooves, undercuts, and fillets. These models serve as the blueprint for the machining process, ensuring that every detail is captured accurately.
- Toolpath Optimization: CAM software converts the 3D CAD model into the G-code used to control the CNC machine. It optimizes the toolpaths to reduce cycle times, minimize tool changes, and ensure smooth transitions between different operations, such as turning, milling, and drilling.
- Simulation and Collision Detection: Before the actual machining process begins, CAM software can simulate the entire machining operation, identifying potential issues such as tool collisions or areas where the part might not meet the required tolerances. This allows manufacturers to refine the process and avoid costly errors during production.
By using modern CAD/CAM software, CNC turning centers can produce highly complex parts while maintaining precision and efficiency. The software provides flexibility in part design and ensures that the machine can handle intricate geometries, minimizing the need for manual intervention or secondary operations.
5. Material Considerations for Complex CNC Turned Parts
When it comes to complex part manufacturing, material selection plays a critical role in determining the feasibility and quality of the finished product. CNC turning is compatible with a wide range of materials, but some materials are better suited for complex geometries due to their machinability and mechanical properties.
- Aluminum: Aluminum is a popular material for complex CNC turning because of its excellent machinability, lightweight properties, and ability to achieve tight tolerances. It is commonly used in aerospace, automotive, and electronics applications where complex geometries and fine details are required.
- Stainless Steel: While stainless steel is more challenging to machine due to its hardness and tendency to work harden, it is still widely used for complex parts that require high strength, corrosion resistance, and durability. It is commonly used in the medical, food processing, and marine industries.
- Titanium: Titanium is highly sought after for its strength-to-weight ratio and corrosion resistance, making it ideal for complex parts in aerospace, medical, and automotive applications. However, it requires specialized tools and cutting techniques due to its hardness and poor thermal conductivity.
- Plastics: Complex parts made from engineering plastics such as PEEK, Delrin, and nylon are common in medical devices, electronics, and consumer goods. These materials are lightweight, resistant to chemicals, and easy to machine, making them suitable for intricate designs with fine details.
- Composites: Advanced composites like carbon fiber-reinforced polymers (CFRP) and glass fiber-reinforced polymers (GFRP) are also used for complex parts, especially in industries where lightweight and high strength are critical. However, machining composites requires specialized tooling to prevent delamination and other defects.
By selecting the appropriate material and ensuring it is compatible with CNC turning processes, manufacturers can produce complex parts that meet both performance and quality requirements.
6. Challenges and Best Practices for Complex CNC Turning
While CNC turning has the capability to produce complex parts, there are several challenges involved in achieving high precision and quality. These challenges include maintaining tight tolerances, managing tool wear, and ensuring efficient production processes. However, with proper planning and execution, these challenges can be overcome.
Challenges:
- Tool Wear: Complex parts often require multiple machining operations, which can increase tool wear, especially when working with hard materials like titanium or stainless steel. Regular monitoring and tool changes are necessary to maintain precision.
- Heat Generation: Complex parts may involve long machining cycles, generating significant heat that can lead to thermal expansion and dimensional inaccuracies. Using appropriate coolant systems and optimizing cutting parameters can mitigate these issues.
- Chip Evacuation: Machining deep features or intricate internal geometries can lead to chip accumulation, which can damage the part or tool. Effective chip evacuation strategies, such as high-pressure coolant systems, are essential for maintaining part quality.
Best Practices:
- Toolpath Planning: Careful planning of toolpaths to minimize tool changes and reduce the likelihood of errors is essential. Multi-axis machines with integrated milling and turning functions can help streamline operations and reduce complexity.
- Use of Simulation: Running simulations before actual production can identify potential issues such as tool collisions or areas of excessive wear. This allows for adjustments before the part is machined, saving time and resources.
- Regular Maintenance: Ensuring that CNC machines are regularly calibrated and maintained is essential for producing complex parts with tight tolerances. This includes checking the alignment of spindles, tool holders, and other critical components.
Is CNC Programming for Turning Difficult?
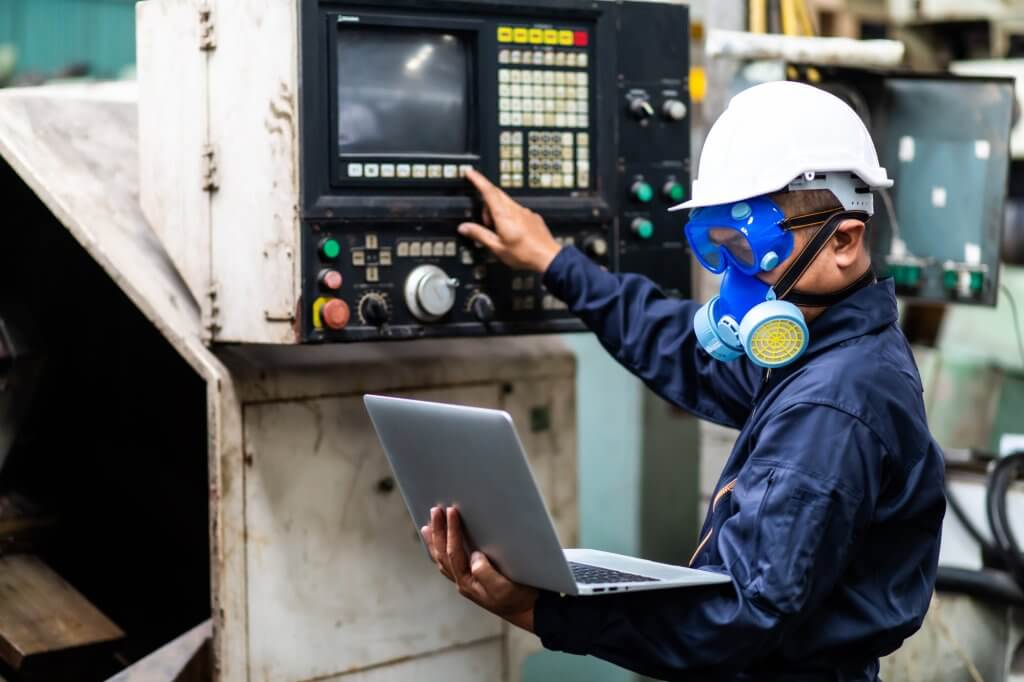
CNC programming for turning can vary in difficulty depending on the complexity of the part, the level of automation in the CNC machine, the programmer’s experience, and the capabilities of the software being used. While CNC turning has evolved to accommodate increasingly complex geometries and tight tolerances, mastering the programming process requires a blend of technical skill, knowledge of machining principles, and experience with specialized software.
This chapter will explore the various factors that contribute to the difficulty of CNC programming for turning, such as machine configurations, tooling choices, material characteristics, and software capabilities. We will also review the learning curve for new programmers and discuss strategies for simplifying the CNC programming process for turning operations.
1. Understanding CNC Programming Basics for Turning
Before diving into the complexities of CNC programming, it’s important to understand the basics. CNC turning is a machining process where a workpiece is rotated, and a cutting tool removes material to shape the workpiece into the desired geometry. CNC programming involves creating a set of instructions, typically in G-code, that tells the CNC machine how to move, at what speed, and how deep to cut to achieve the desired part dimensions.
Some of the fundamental concepts in CNC turning programming include:
- Axes of Movement: CNC turning machines typically operate on two axes: the X-axis (which controls the movement of the cutting tool towards or away from the workpiece’s center) and the Z-axis (which controls the movement of the cutting tool along the length of the rotating workpiece). In more advanced machines, additional axes such as Y-axis and C-axis are introduced to allow for more complex operations.
- Cutting Parameters: These include spindle speed, feed rate, and depth of cut. The spindle speed controls the rotation of the workpiece, while the feed rate dictates how fast the cutting tool moves. The depth of cut determines how much material is removed in a single pass.
- Toolpath: The toolpath refers to the route that the cutting tool takes as it removes material from the workpiece. In CNC turning, the toolpath is primarily defined by the shape and dimensions of the final part.
- G-Code: G-code is the language that CNC machines use to interpret programming instructions. Each line of G-code provides specific information to the machine, such as where to move, how fast to rotate, and when to engage the cutting tool.
While the basics of CNC programming may seem straightforward, the difficulty increases as the complexity of the part and the machining operations increase. For simple parts with basic geometries, programming is relatively easy. However, when dealing with intricate shapes, tight tolerances, and multi-axis machines, programming becomes more challenging.
2. Factors That Contribute to the Complexity of CNC Turning Programming
Several factors contribute to the complexity of CNC programming for turning, and understanding these factors can help assess whether CNC turning is difficult for a given project. Here are some of the key challenges:
2.1. Part Geometry
The geometry of the part being machined is one of the primary factors determining the difficulty of CNC programming. Simple parts with cylindrical or symmetrical features are relatively easy to program. However, complex parts with non-cylindrical features, multiple radii, undercuts, or tight tolerances require more sophisticated programming techniques.
- Symmetrical vs. Asymmetrical Parts: Symmetrical parts, such as simple shafts or bolts, follow predictable toolpaths, making them easier to program. Asymmetrical parts or parts with complex profiles, such as turbine blades, require intricate toolpath planning and often demand multi-axis machines, increasing programming difficulty.
- Internal Features: Parts with internal features such as bores, threads, or internal grooves add complexity to the programming. The cutting tool must be carefully positioned, and the toolpath must be planned to avoid collisions or tool interference within the internal geometry.
- Multi-Feature Parts: Parts that require a combination of turning, milling, drilling, and threading operations add complexity to the programming process, as each operation needs to be carefully coordinated within the same program.
2.2. Machine Capabilities
The capabilities of the CNC turning machine itself also influence programming difficulty. Modern turning centers come with a variety of features that can make programming either more straightforward or more complex, depending on how advanced the machine is.
- Multi-Axis Machines: CNC turning centers equipped with additional axes (such as the Y-axis or C-axis) allow for more complex operations, such as off-center drilling, contour milling, or creating intricate part features. However, programming these machines requires a deeper understanding of multi-axis machining principles and advanced software.
- Live Tooling: Machines with live tooling capabilities enable turning centers to perform milling, drilling, and other operations without removing the part from the spindle. This reduces the need for multiple setups but introduces additional complexity into the programming process, as the programmer must account for the orientation and movement of the live tools.
- Sub-Spindle Operations: Some CNC turning centers are equipped with a sub-spindle, allowing the part to be transferred from the main spindle to the sub-spindle for machining on the opposite side. This feature eliminates manual repositioning, but it adds complexity to the programming since it requires synchronization between the two spindles.
2.3. Material Considerations
The material being machined also affects the difficulty of CNC programming for turning. Different materials have different machinability properties, requiring adjustments in cutting parameters and tool selection. Some materials are easier to machine, while others pose significant challenges.
- Soft Materials: Materials such as aluminum or plastics are relatively easy to machine, making the programming less difficult. The cutting speeds and feed rates can be higher, and tool wear is less of a concern.
- Hard Materials: Materials such as titanium, hardened steel, and superalloys are more difficult to machine due to their hardness and heat resistance. CNC programming for these materials requires more careful consideration of cutting speeds, feed rates, and toolpaths to prevent tool breakage and ensure accurate cuts.
- Composite Materials: Composites such as carbon fiber-reinforced polymers (CFRP) require specialized tooling and machining strategies to prevent delamination or fiber damage. CNC programming for these materials is more challenging because of the need for optimized toolpaths and tool choices to reduce the risk of defects.
2.4. Tool Selection and Management
Choosing the right cutting tools for CNC turning is critical for achieving the desired precision and surface finish. Tool selection can significantly impact the complexity of the programming process. Factors to consider include tool material, geometry, and coating, all of which affect the tool’s performance and wear resistance.
- Tool Types: Various types of cutting tools, such as boring bars, drills, threading tools, and grooving tools, are used in CNC turning. The programmer must ensure that each tool is selected appropriately for the specific feature being machined and that tool changes are minimized to reduce cycle time.
- Tool Wear: Tool wear is a common issue in CNC turning, especially when machining hard materials or using high cutting speeds. Programmers must account for tool wear and program the machine to periodically check for wear or change tools as needed. This requires adding tool wear compensation to the program and optimizing the toolpath to extend tool life.
2.5. Achieving Tolerances and Surface Finish
For many CNC-turned parts, achieving tight tolerances and fine surface finishes is critical. These requirements can add a layer of complexity to the programming process.
- Tight Tolerances: Parts with tight tolerances require careful control of tool positioning and cutting parameters to ensure that dimensions are within specified limits. Achieving tight tolerances may require multiple finishing passes, which must be programmed carefully to avoid over-cutting or tool deflection.
- Surface Finish: Achieving a fine surface finish often requires the use of specialized cutting tools, slower feed rates, and optimized toolpaths. Programmers must account for factors such as tool vibration, chatter, and heat generation, which can affect the final surface quality.
3. CNC Programming Software for Turning
The difficulty of CNC programming is greatly influenced by the software tools available. Modern CNC programming software has evolved to make the process easier and more intuitive, even for complex parts. The two main categories of software used for CNC turning programming are CAM software and machine control software.
3.1. CAM Software
Computer-Aided Manufacturing (CAM) software plays a central role in simplifying the CNC programming process. CAM software allows engineers and machinists to create toolpaths, generate G-code, and simulate machining operations before they are executed on the CNC machine. Some popular CAM software programs used in CNC turning include Mastercam, Fusion 360, and Siemens NX.
Key features of CAM software that reduce programming difficulty include:
- Automatic Toolpath Generation: CAM software can automatically generate toolpaths based on the part geometry, significantly reducing the time and effort needed to program complex parts. This feature is especially useful for multi-axis machines or parts with intricate geometries.
- Simulation and Verification: CAM software includes simulation tools that allow programmers to visualize the machining process and check for potential issues such as tool collisions, excessive tool wear, or out-of-tolerance features. This helps reduce the risk of errors during the actual machining process.
- Optimization Tools: CAM software can optimize toolpaths to reduce cycle time and improve efficiency. Features like high-speed machining (HSM) and adaptive toolpaths help minimize tool wear and improve the overall quality of the part.
3.2. Machine Control Software
Once the G-code is generated using CAM software, it must be transferred to the CNC machine’s control software. The control software interprets the G-code and executes the machining operations. Some advanced CNC machines have user-friendly control interfaces that make it easier to fine-tune the program and adjust parameters in real time.
–
Real-Time Adjustments: Advanced machine control software allows operators to make real-time adjustments to cutting parameters such as spindle speed, feed rate, and depth of cut. This can be helpful when dealing with difficult-to-machine materials or parts that require tight tolerances.
- Macro Programming: Many CNC control systems support macro programming, which allows for the creation of reusable code blocks for common machining tasks. This can simplify programming for parts that share similar features, reducing the time and effort needed to program each part individually.
4. The Learning Curve for CNC Turning Programming
For individuals new to CNC turning, the learning curve can be steep, especially when dealing with complex parts and multi-axis machines. However, with the right training and practice, CNC programming for turning can become more manageable.
- Understanding Machining Principles: A strong foundation in machining principles is essential for learning CNC programming. This includes knowledge of cutting tool geometry, material properties, and machining processes such as turning, threading, drilling, and milling.
- Mastering G-Code: G-code is the backbone of CNC programming, and new programmers must learn how to write, read, and interpret G-code effectively. While CAM software can generate G-code automatically, understanding the language is still critical for troubleshooting and making manual adjustments.
- Hands-On Practice: Learning CNC programming requires hands-on practice with actual machines. Many CNC programming courses offer access to CNC simulators or real machines where students can practice writing programs and running parts.
- Continuous Learning: CNC programming is a constantly evolving field, with new software tools, machining techniques, and technologies being introduced regularly. To stay competitive, CNC programmers must continuously update their skills and stay informed about the latest advancements in the industry.
5. Best Practices for Simplifying CNC Turning Programming
While CNC programming for turning can be complex, there are several best practices that can simplify the process and improve efficiency:
- Standardize Tooling: Using standardized tooling and fixtures across multiple parts can reduce the time needed to program each part individually. By developing a consistent tool library and using the same tools for similar operations, programmers can streamline the process.
- Use Templates: Many CAM software programs allow users to create templates for common machining operations, such as roughing, finishing, and threading. These templates can be reused for similar parts, reducing programming time.
- Optimize Toolpaths: Optimizing toolpaths to minimize tool changes and reduce cutting time can improve both productivity and part quality. Using CAM software’s toolpath optimization features can help achieve this.
- Simulate First: Always simulate the machining process before running the program on the CNC machine. This helps identify potential issues such as tool collisions or areas of excessive wear, reducing the risk of errors during production.
- Leverage Multi-Axis Capabilities: For complex parts, using multi-axis machines with integrated milling and turning functions can reduce the number of setups required and simplify the programming process.
How to Choose the Right CNC Turning Tools?
Choosing the right tools for CNC turning is a critical aspect of achieving high precision, efficiency, and cost-effectiveness in machining operations. With numerous factors influencing the selection process—such as material properties, part geometry, machine capabilities, and desired surface finish—the proper selection of turning tools can greatly enhance the quality of the final product while minimizing tool wear, cycle time, and production costs.
This chapter explores the key considerations when selecting CNC turning tools, covering various tool types, material compatibility, cutting parameters, tool geometry, and coatings. Additionally, we will discuss how modern tool management systems can aid in making informed decisions to optimize machining processes.
1. Understanding CNC Turning Tool Types
CNC turning operations require a variety of tools to perform specific tasks such as cutting, shaping, drilling, and finishing. The right tool must be selected based on the specific requirements of the part being machined.
1.1. Common Types of CNC Turning Tools
- Turning Tools: These are the primary tools used in CNC turning operations. They include roughing and finishing tools that shape the exterior of cylindrical workpieces.
- Roughing Tools: Used for the initial phase of turning to remove large amounts of material quickly. They have a large depth of cut and are designed for high material removal rates but may leave a rough surface.
- Finishing Tools: Used after roughing tools to refine the workpiece and achieve the final dimensions and surface finish. Finishing tools have a shallower depth of cut and are used at lower speeds to produce smoother surfaces.
- Parting (Cut-Off) Tools: Used to separate (part off) the finished part from the raw material or to create grooves. The tool has a narrow blade that cuts through the workpiece perpendicular to the axis of rotation.
- Grooving Tools: Designed to create internal or external grooves on a workpiece. They typically have specially shaped cutting edges for producing the desired groove geometry.
- Threading Tools: Used to cut internal or external threads on a workpiece. Threading tools must be precisely designed to match the thread profile and pitch required by the part.
- Boring Tools: Used for enlarging or finishing internal cylindrical holes in a workpiece. They are critical when creating high-precision bores with tight tolerances.
- Drilling Tools: While drilling is often associated with CNC milling, drilling tools are also used in turning operations, particularly for creating holes in the center of the rotating workpiece.
1.2. Tool Inserts and Indexable Inserts
Tool inserts are replaceable cutting tips attached to the toolholder. These inserts come in a variety of shapes and materials to suit different machining conditions. Indexable inserts are widely used because they allow the tool to rotate to a new cutting edge when one side becomes dull, reducing downtime and improving tool life.
- Common Insert Shapes:
- Round Inserts: Provide multiple cutting edges, ideal for roughing and large depths of cut.
- Square Inserts: Often used for heavy-duty turning with good resistance to impact and wear.
- Triangular Inserts: Versatile inserts used in both roughing and finishing operations.
- Diamond Inserts: Used for finishing operations requiring high precision and smooth surface finishes.
2. Material Considerations in Tool Selection
The choice of cutting tool material is influenced by the material being machined, as different workpiece materials have unique properties such as hardness, toughness, and machinability. The material of the tool determines its cutting performance, wear resistance, and longevity.
2.1. Common Tool Materials
- High-Speed Steel (HSS): Known for its toughness and ability to withstand high cutting forces, HSS is commonly used in turning tools for lower-speed applications and softer materials like aluminum and plastics. HSS tools are typically less expensive but wear out faster than other materials.
- Carbide: Widely used in CNC turning due to its excellent hardness and wear resistance, carbide can maintain sharp cutting edges at high temperatures, making it suitable for high-speed machining of harder materials such as stainless steel, cast iron, and titanium.
- Ceramic: Used for high-speed turning applications involving hard materials, ceramic is resistant to wear and can withstand high cutting temperatures, but it is more brittle than carbide and susceptible to chipping.
- Cermet: A hybrid material combining ceramic and metal, cermet inserts provide a balance between the toughness of metal and the hardness of ceramic. They are often used for high-precision finishing operations, especially when a smooth surface finish is required.
- Cubic Boron Nitride (CBN): Used for turning hard materials like hardened steel and nickel-based alloys, CBN provides extreme hardness and wear resistance but is expensive, making it ideal for finishing operations where precision is critical.
- Diamond: Polycrystalline diamond (PCD) tools are the hardest cutting tools available, ideal for machining non-ferrous materials like aluminum, copper, and composite materials. PCD tools offer excellent wear resistance and can achieve superior surface finishes but are expensive and limited to specific materials.
2.2. Workpiece Material Considerations
The machinability of different materials varies, and the choice of tool must account for these differences to optimize performance.
Material Type | Preferred Tool Material | Machining Considerations |
---|---|---|
Aluminum & Alloys | Carbide, HSS, Diamond (PCD) | High cutting speeds, avoid excessive built-up edge, ensure excellent surface finish |
Stainless Steel | Carbide, CBN | Moderate cutting speeds, avoid work hardening, requires sharp tools and controlled temperatures |
Titanium & Superalloys | Carbide, CBN, Ceramic | Low cutting speeds, avoid tool deflection, use of rigid setups and efficient cooling required |
Cast Iron | Carbide, Ceramic | High wear resistance needed, avoid excessive vibration, use of interrupted cuts may be required |
Plastics & Composites | Carbide, HSS, Diamond (PCD) | Low cutting forces, avoid melting, ensure excellent surface finish, reduce tool friction |
Hardened Steel | CBN, Ceramic, Carbide | High hardness requires wear-resistant tools, slow cutting speeds, avoid excessive heat buildup |
3. Tool Geometry: Critical Factors in Performance
The geometry of the cutting tool is another crucial aspect to consider when selecting turning tools. Tool geometry affects how the tool engages with the workpiece, influences chip formation, and impacts overall machining performance.
3.1. Tool Geometry Elements
- Rake Angle: The rake angle is the angle formed between the tool’s cutting edge and the workpiece surface. It affects the ease with which the tool cuts through the material. Positive rake angles reduce cutting forces and improve surface finish, while negative rake angles are more durable and can handle higher cutting forces.
- Relief Angle: The relief angle is the angle formed between the flank of the tool and the surface being cut. It ensures that the tool does not rub against the workpiece, which can lead to poor surface finish and tool wear.
- Cutting Edge Radius: The cutting edge radius, also known as the nose radius, is the rounded tip of the tool where the cutting edge meets the workpiece. A larger nose radius improves surface finish and tool strength but requires higher cutting forces, while a smaller radius is used for fine finishing operations.
3.2. Matching Tool Geometry to the Operation
- Roughing Operations: Tools used for roughing operations typically have a larger nose radius and a more durable geometry to withstand high material removal rates. Negative rake angles are often used to handle the higher cutting forces.
- Finishing Operations: For finishing operations, tools with a smaller nose radius and positive rake angles are preferred. These tools are designed to produce smooth surface finishes and hold tight tolerances.
- Threading and Grooving: Special geometries are required for threading and grooving tools to match the specific profiles of the threads or grooves being cut.
4. Tool Coatings: Enhancing Performance and Longevity
Tool coatings play an important role in improving tool life and enhancing cutting performance. Different coatings offer protection against wear, heat, and oxidation, making them suitable for specific applications.
- Titanium Nitride (TiN): TiN coatings are commonly used on carbide tools to reduce friction and improve wear resistance. TiN is ideal for general-purpose machining operations, especially in materials like steel and aluminum.
- Titanium Carbonitride (TiCN): TiCN coatings provide improved hardness and wear resistance over TiN. They are well-suited for machining harder materials such as stainless steel and cast iron.
- Aluminum Titanium Nitride (AlTiN): AlTiN coatings offer excellent resistance to heat and oxidation, making them ideal for high-speed machining of hard materials like titanium and nickel alloys.
- Diamond Coatings: Diamond coatings, particularly in the form of PCD, are ideal for non-ferrous materials and composites. They provide excellent wear resistance and can achieve superior surface finishes.
5. Cutting Parameters and Their Influence on Tool Selection
The choice of cutting parameters—such as spindle speed, feed rate, and depth of cut—has a significant influence on tool selection. The correct parameters optimize machining efficiency while minimizing tool wear and improving part quality.
5.1. Spindle Speed
The spindle speed determines how fast the workpiece rotates during the turning process. For softer materials like aluminum, higher spindle speeds are appropriate, whereas for harder materials, lower spindle speeds are required to prevent excessive tool
wear.
5.2. Feed Rate
The feed rate controls how fast the cutting tool moves relative to the workpiece. A higher feed rate can reduce cycle time but may compromise surface finish. For finishing operations, lower feed rates are typically used to achieve better surface quality.
5.3. Depth of Cut
The depth of cut refers to how much material is removed in one pass of the cutting tool. Larger depths of cut are typically used in roughing operations, while shallow cuts are used in finishing to achieve precise dimensions and smooth surfaces.
6. Tool Management Systems for CNC Turning
As CNC turning operations grow in complexity, the use of tool management systems becomes essential for maximizing efficiency and reducing downtime. These systems track tool usage, predict wear, and help schedule tool maintenance or replacements.
- Tool Tracking: Tool management systems keep records of tool life, usage history, and wear conditions. This helps prevent unexpected tool failure and ensures that tools are replaced before they reach critical wear levels.
- Tool Presetting: Presetting tools before they are used in machining allows for faster changeovers and minimizes machine downtime. Tool management systems store preset parameters, making tool setup quicker and more accurate.
- Tool Inventory Management: By tracking tool usage across multiple machines, tool management systems help maintain optimal inventory levels, ensuring that the right tools are available when needed without overstocking.
By carefully considering these factors and implementing effective tool management practices, manufacturers can optimize their CNC turning operations and achieve high-quality results in their machining processes.
How to Evaluate the Efficiency and Cost of CNC Turning?
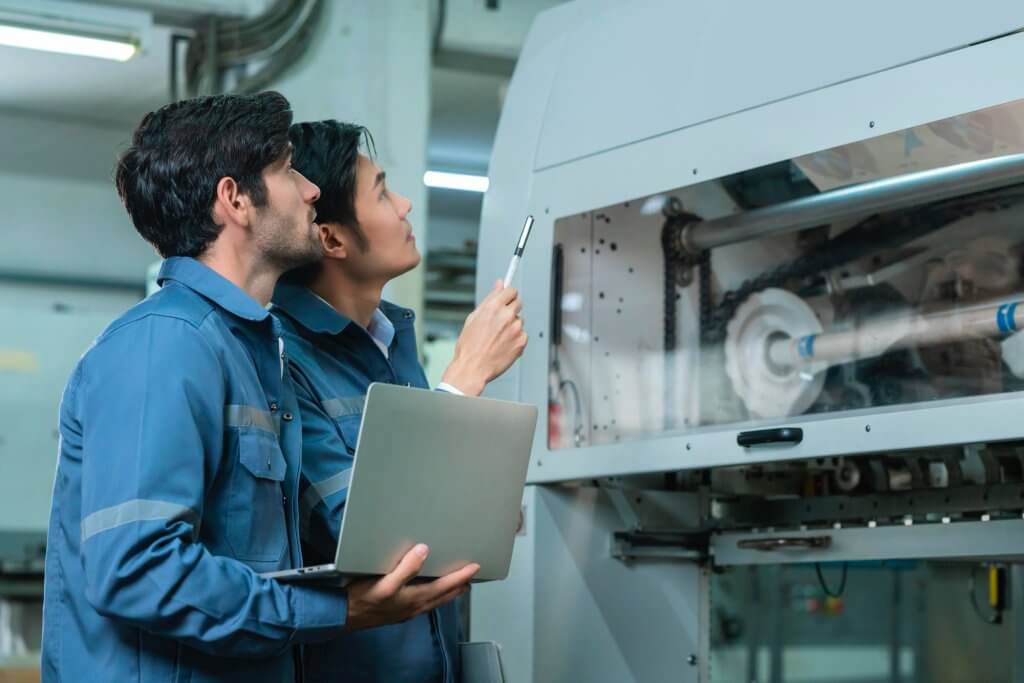
Evaluating the efficiency and cost of CNC turning is essential for optimizing manufacturing processes, improving productivity, and controlling expenses. This assessment involves understanding various factors such as machine utilization, material selection, tool life, cutting parameters, and labor. By accurately measuring these aspects, manufacturers can make informed decisions to maximize production output while minimizing waste and costs.
This chapter explores the key factors influencing the efficiency and cost of CNC turning, how to measure them effectively, and strategies to enhance both. We will also examine how modern technological advancements and software solutions can streamline the evaluation process.
1. Key Factors Affecting the Efficiency of CNC Turning
Efficiency in CNC turning refers to the ability to produce quality parts in the shortest possible time while minimizing resource consumption. Several factors contribute to the overall efficiency of CNC turning, including machine utilization, cutting tool performance, cycle time, and the accuracy of machining operations.
1.1. Machine Utilization
Machine utilization is a measure of how effectively the CNC machine is used during production. It is critical to maximizing efficiency and reducing idle time. Low machine utilization means that the machine is not being used to its full potential, leading to increased overhead costs.
- Idle Time: Idle time occurs when the machine is not producing parts, such as during setup changes, tool changes, or maintenance. Reducing idle time through optimized scheduling, automatic tool changers (ATC), and efficient setup processes can significantly improve efficiency.
- Downtime: Downtime can result from machine breakdowns, maintenance issues, or unplanned stoppages. Regular preventive maintenance, predictive diagnostics, and real-time monitoring of machine health can reduce downtime and improve machine availability.
1.2. Cycle Time
Cycle time refers to the total time taken to produce one part, including all machining operations, tool changes, and any additional processes like cleaning or inspection. Reducing cycle time is one of the most direct ways to improve efficiency.
Factors that affect cycle time include:
- Spindle Speed and Feed Rate: These are critical cutting parameters that influence material removal rates. Optimizing spindle speed and feed rate for the material and tool in use can minimize cycle time without sacrificing quality.
- Tool Changes: Minimizing the number of tool changes or using multi-functional tools can reduce cycle time. Advanced CNC machines with ATC systems can also expedite tool changes.
- Part Handling: Efficient part loading and unloading, either manually or using automation, can reduce the overall cycle time.
1.3. Tool Life
Tool life has a direct impact on both efficiency and cost. Longer tool life reduces the frequency of tool changes, leading to fewer interruptions in production. However, running a tool for too long can result in tool failure, poor surface quality, and costly rework or scrap.
- Cutting Parameters: Adjusting spindle speed, feed rate, and depth of cut can optimize tool life. Running tools at their optimal conditions prevents premature wear and maximizes tool efficiency.
- Tool Wear Monitoring: Advanced systems that monitor tool wear and provide alerts when tools need to be replaced can enhance tool life and prevent unexpected breakdowns.
- Tool Coatings and Material: Using the right tool materials and coatings for specific materials can extend tool life and improve cutting performance. For example, carbide and coated tools typically last longer than HSS tools in high-speed operations.
1.4. Part Complexity and Geometry
The complexity of the part being machined plays a significant role in determining the efficiency of CNC turning. More intricate geometries or tight tolerances often require slower cutting speeds, multiple passes, or special tooling, all of which increase cycle time.
- Complex Features: Parts with complex features such as undercuts, threads, or deep bores require more advanced machining strategies and may involve multiple setups or specialized tools, reducing overall efficiency.
- Multi-Axis Machining: For complex parts, multi-axis CNC machines can reduce the number of setups required and allow for more efficient machining. However, programming and setup for multi-axis machines are typically more complex and time-consuming.
1.5. Material Selection
The choice of material also affects machining efficiency. Different materials have varying degrees of machinability, which impacts cutting speeds, tool wear, and overall cycle time.
- High Machinability Materials: Materials like aluminum and brass are easier to machine, allowing for higher cutting speeds and lower tool wear, thereby improving efficiency.
- Low Machinability Materials: Harder materials like titanium, Inconel, or stainless steel require slower cutting speeds, frequent tool changes, and more conservative machining parameters, which can reduce efficiency.
2. Key Factors Affecting the Cost of CNC Turning
Cost evaluation in CNC turning involves both direct and indirect costs. Direct costs include material, tooling, machine time, and labor, while indirect costs cover overhead, maintenance, and administrative expenses. Reducing these costs while maintaining high production standards is crucial for profitability.
2.1. Material Costs
Material cost is one of the primary expenses in CNC turning operations. It is important to choose materials that are both cost-effective and suitable for the part’s requirements.
- Raw Material Costs: The cost of raw materials can vary significantly depending on the type of material, the form (bars, billets, or sheets), and the quantity purchased. Bulk purchasing can lower costs, but it requires accurate forecasting and inventory management.
- Material Waste: Reducing material waste through optimized cutting strategies and nesting can lower material costs. Efficient programming can ensure that the maximum amount of material is used with minimal scrap.
2.2. Tooling Costs
Tooling costs include the purchase of cutting tools, tool holders, and fixtures, as well as costs associated with tool wear and replacement.
- Tooling Selection: Using high-quality, durable tools may have a higher upfront cost, but they often provide better performance, longer tool life, and reduced downtime, leading to lower overall costs.
- Tool Regrinding and Reuse: Regrinding worn tools rather than replacing them with new ones can help reduce tooling costs. This is especially important for expensive tools like carbide or diamond-coated tools.
- Tool Inventory Management: Proper tool inventory management helps reduce over-purchasing or understocking, both of which can negatively impact cost and production efficiency.
2.3. Machine Time and Labor Costs
Machine time and labor costs are closely related to production efficiency. Reducing cycle time, minimizing downtime, and optimizing machine utilization all contribute to lowering machine time and labor expenses.
- Operator Skill Level: Skilled operators can improve the efficiency of CNC turning operations by optimizing tool selection, adjusting cutting parameters, and reducing setup time. Investing in operator training can lead to long-term cost savings.
- Automation: Automated CNC machines with robotic part handling or pallet changers can significantly reduce labor costs by minimizing the need for manual intervention. Automation also improves production speed and consistency.
2.4. Maintenance and Overhead Costs
Routine machine maintenance is essential for preventing unexpected breakdowns and minimizing repair costs. However, maintenance schedules and procedures need to be balanced with production demands to avoid excessive downtime.
- Preventive Maintenance: Regular preventive maintenance helps extend the life of CNC machines, ensuring consistent performance and reducing the likelihood of costly repairs. Maintaining machine cleanliness, lubrication, and alignment are critical to preventing unexpected failures.
- Predictive Maintenance: Advanced predictive maintenance systems use sensors and real-time data to predict potential machine issues before they occur. By addressing problems early, manufacturers can avoid unplanned downtime and costly repairs.
- Overhead Costs: Overhead costs, such as utilities, facility maintenance, and administrative expenses, must also be factored into the total cost of CNC turning operations. Efficient energy use and resource management can help reduce overhead.
3. Measuring Efficiency and Cost in CNC Turning
To accurately evaluate the efficiency and cost of CNC turning, manufacturers must adopt measurement and tracking systems. Key performance indicators (KPIs) and cost metrics provide valuable insights into how well a production process is functioning and where improvements can be made.
3.1. Key Performance Indicators (KPIs) for Efficiency
Tracking KPIs related to CNC turning efficiency helps manufacturers identify bottlenecks, optimize production, and improve overall performance. Common KPIs include:
- Overall Equipment Effectiveness (OEE): OEE measures how effectively a CNC machine is being utilized, combining availability, performance, and quality metrics into a single figure. A high OEE score indicates that the machine is operating at optimal efficiency.
OEE Component | Formula |
---|---|
Availability | Run Time / Total Time |
Performance | (Ideal Cycle Time * Total Parts) / Run Time |
Quality | Good Parts / Total Parts |
- Cycle Time: This metric measures the time taken to produce a single part. Reducing cycle time directly improves production throughput and efficiency.
- Tool Change Time: Monitoring how long it takes to change tools can help identify areas where efficiency can be improved, such as through faster tool changers or tool pre-setting.
- Downtime: Tracking machine downtime helps identify common causes, such as machine failures, maintenance, or setup delays, so corrective actions can be implemented.
3.2. Cost Metrics for CNC Turning
To evaluate the cost of CNC turning, manufacturers should track various cost-related metrics that impact profitability.
- Cost Per Part: This metric helps assess the overall cost of producing each part and includes material, tooling, labor, and machine time costs. By lowering the cost per part, manufacturers can improve margins.
Cost Factor | Example Calculation |
---|---|
Material Cost | Total Material Used * Material Price |
Tooling Cost | Tool Purchase Cost / |
Number of Parts Produced |
| Machine Time Cost | Machine Hourly Rate * Cycle Time |
| Labor Cost | Labor Hourly Rate * Labor Time |
- Tooling Cost Per Part: Dividing the total tooling cost by the number of parts produced helps assess the impact of tooling expenses on overall costs. Reducing tool wear and optimizing tool life are essential for lowering this metric.
- Scrap and Rework Costs: Tracking the costs associated with scrap and rework helps identify inefficiencies in the process. Reducing defects and improving quality control measures can minimize these costs.
4. Strategies to Improve Efficiency and Reduce Costs in CNC Turning
Improving efficiency and reducing costs in CNC turning requires a combination of technological advancements, process optimization, and workforce training.
4.1. Implementing Automation
Automation solutions such as robotic arms, automatic part loaders, and pallet changers can significantly reduce cycle time and labor costs. Automation also improves consistency, reduces the risk of human error, and allows for lights-out machining—where machines run unsupervised during off-hours.
4.2. Optimizing Cutting Parameters
Optimizing spindle speeds, feed rates, and depth of cut can improve tool life, reduce cycle time, and enhance surface finish. Using modern CAM software to simulate and test different cutting strategies can help find the most efficient parameters.
4.3. Reducing Tool Changes
Using multi-functional tools or reducing the number of tool changes during a cycle can improve efficiency. Tools that combine turning, threading, and grooving operations in one can help streamline the process.
4.4. Investing in Skilled Labor
Training operators to use CNC machines more efficiently, make real-time adjustments, and troubleshoot issues can significantly enhance overall productivity. Skilled operators can also optimize machine setups, reduce cycle times, and improve tool life through better parameter management.
4.5. Advanced Monitoring and Maintenance Systems
Implementing advanced machine monitoring systems allows manufacturers to track machine performance, predict tool wear, and detect anomalies. Predictive maintenance systems can minimize downtime by addressing issues before they lead to machine failure.
FAQ
1. What is the difference between CNC turning and CNC lathe machines?
CNC turning refers to the actual machining process where a workpiece is rotated while a cutting tool removes material. A CNC lathe machine is the specific machine used to perform CNC turning. Essentially, CNC turning is the operation, while a CNC lathe is the tool that carries out that operation.
2. What industries commonly use CNC turning?
CNC turning is widely used in industries such as aerospace, automotive, medical devices, electronics, and heavy machinery. It is essential for producing precision components such as shafts, gears, bushings, and fasteners.
3. How accurate is CNC turning?
CNC turning is highly accurate, capable of achieving tolerances as tight as ±0.0005 inches (0.0127 mm) or better. This level of precision is ideal for manufacturing parts that require exact measurements, such as engine components, medical instruments, and aerospace parts.
4. How does tool wear affect CNC turning operations?
Tool wear can significantly impact CNC turning operations by reducing the quality of the surface finish, increasing cutting forces, and causing dimensional inaccuracies. Monitoring tool wear and replacing tools before they fail is essential for maintaining part quality and reducing downtime.
5. Can CNC turning be used for mass production?
Yes, CNC turning is highly suitable for mass production, particularly when producing components with consistent geometries. CNC machines are programmable and repeatable, making them ideal for manufacturing large quantities of parts efficiently.
6. What materials can be processed with CNC turning?
CNC turning can process a wide range of materials, including metals like aluminum, steel, brass, and titanium, as well as plastics, composites, and exotic alloys. Material choice depends on the specific requirements of the part being machined, such as strength, weight, and thermal properties.
7. How does CNC turning compare to manual turning?
CNC turning offers significantly higher precision, repeatability, and speed compared to manual turning. It allows for complex part geometries to be programmed and automatically machined, reducing human error and labor costs. Manual turning, while still useful, is better suited for low-volume or simple parts.
8. How does CNC turning handle complex geometries?
CNC turning can handle complex geometries through advanced multi-axis CNC lathes. These machines can perform multiple operations in a single setup, such as contouring, grooving, and threading, allowing for the efficient machining of parts with intricate designs.
9. What role does coolant play in CNC turning?
Coolant helps to reduce heat generated during the turning process, protecting the cutting tool and the workpiece from thermal damage. It also aids in chip removal and improves surface finish by reducing friction between the tool and the material.
10. How do I maintain a CNC lathe machine?
To maintain a CNC lathe machine, regular preventive maintenance should be performed. This includes cleaning, lubrication, inspecting tool wear, checking alignment, and monitoring machine accuracy. A well-maintained machine ensures consistent performance and reduces unexpected breakdowns.
11. What are common causes of defects in CNC turning?
Common causes of defects in CNC turning include incorrect tool selection, improper cutting parameters, tool wear, machine misalignment, and poor material quality. These issues can result in poor surface finish, dimensional inaccuracies, or part rejection.
12. How does CNC turning contribute to sustainable manufacturing?
CNC turning contributes to sustainable manufacturing by minimizing material waste and energy consumption through optimized cutting paths and efficient machine operation. Additionally, CNC machines can be automated to reduce labor and lower carbon footprints, contributing to greener production processes.
13. How is CNC turning programmed?
CNC turning is programmed using G-code, a standardized programming language that instructs the machine on how to move, what speed to operate at, and where to position the tool. Modern CAM software helps generate G-code from CAD models, simplifying the programming process.
14. Can CNC turning produce both internal and external features?
Yes, CNC turning can produce both internal and external features on a workpiece. External operations include turning, facing, and threading, while internal operations can involve boring, drilling, and reaming.
15. How do I choose the right cutting tool for CNC turning?
The right cutting tool for CNC turning depends on factors such as the workpiece material, required surface finish, cutting speed, and tool durability. Common tool materials include high-speed steel (HSS), carbide, and ceramic, with specific coatings used to enhance performance.
Other Articles You Might Enjoy
- CNC Turning: A Deep Dive into Rivet Production(cnc laser cutting Adolph)
Introduction:Computer numerical control (CNC) represents one of the most crucial inventions in manufacturing technology. It pertains to a method used to control an array of complex machinery using computer programs…
- Summary of CNC Turning Tool Knowledge
Insert Shapes and Naming Standards International Insert Naming Standards The naming of CNC turning inserts follows international standards, which mainly include the shape, material, and suitable machining conditions of the…
- Reducing Manufacturing Costs with Multi-Material CNC Machining Strategies
Introduction to Manufacturing Costs and CNC Machining Solutions Manufacturing costs significantly impact businesses, encompassing expenses related to materials, labor, and operations. These costs determine the final price of products, affecting…
- Effective CNC Turning and Uses of Different Rivets( plasma cutting Alvin)
Computer Numerical Control (CNC) turning process is an essential part of the industrial manufacturing sector. Its efficiency, precision, and wide application in various fields make it a vital production tool.…
- Transforming the Marine Industry with Precision CNC Machined Parts
Introduction to CNC Machining and its Application in the Marine Industry CNC machining, which stands for Computer Numerical Control machining, is a manufacturing process where pre-programmed computer software dictates the…
- Online CNC Machining Service for Stainless Steel Components
Introduction to Online CNC Machining Services for Stainless Steel Components CNC (Computer Numerical Control) machining is a manufacturing process wherein pre-programmed computer software directs the movement of factory machines and…